Biomimetics in Textiles: A Deeper Look
The term biomimetics, which was coined by Otto H Schmitt (Schmitt 1969), represents the studies and imitation of nature’s methods, mechanisms and processes. Nature’s capabilities are far superior in many areas to human capabilities, and adapting many of its features and characteristics can significantly improve our technology [1].
Biomimetics is derived from the Greek word biomimesis. The word was coined by polymath Otto Schmitt in 1957, who, in his doctoral research, developed a physical device that mimicked the electrical action of a nerve. Other words used include bionics, biomimicry and biognosis.
Definition Given by Webster’s DictionaryThe word biomimetics first appeared in Webster’s dictionary in 1974 and is defined as ‘the study of the formation, structure or function of biologically produced substances and materials (as enzymes or silk) and biological mechanisms and processes (as protein synthesis or photosynthesis) especially for the purpose of synthesizing similar products by artificial mechanisms which mimic natural ones’ [2].
Flying was inspired by birds using human-developed capabilities, whereas the design and function of fins, which divers use, was copied from the legs of water creatures like seals. Once human flying became feasible, improvements in aircraft technology led to capabilities that far exceed any creature living on earth (see the example in figure 1).
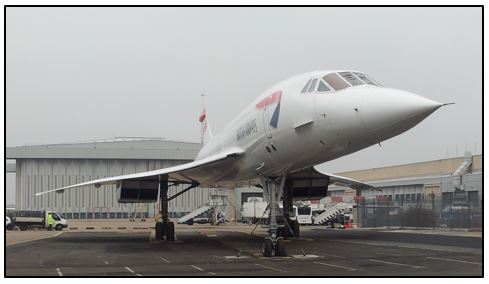
Figure 1: The desire to fly was implemented using aerodynamic principles leading to enormous capabilities such as the supersonic passenger plane, the Concorde
What is Supersonic Speed?
Plants also offer ideas for imitation and they have evolved in various ways, with some that produced uncommon solutions to their special needs. In addition to their familiar characteristics, some plants exhibit actuation capabilities that we would expect from biological creatures. Such plants include mimosa and sensitive fern (onoclea sensibilis) that bend their leaves when touched (see figure 2).
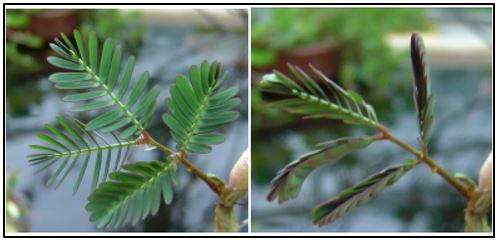
Figure 2: The sensitive fern has its leaves open (left) until they are touched (right)
There are also bug-eating plants with a leaf-derived trap that closes the ‘door’ locking unsuspecting bugs that enter the cage and become prey (see figure 3).
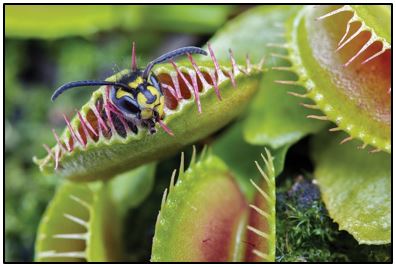
Figure 3: Bug Eating Plant (Venus flytrap)
The sunflower tracks the sun’s direction throughout the day to maximize exposure to its light [1].
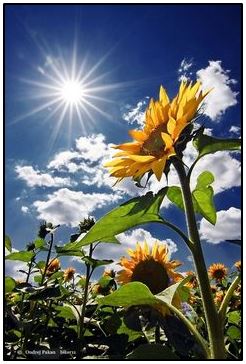
Figure 4: Sunflower tracking the sun’s direction
Overview of Various Objects from Nature & Their Selected Functions
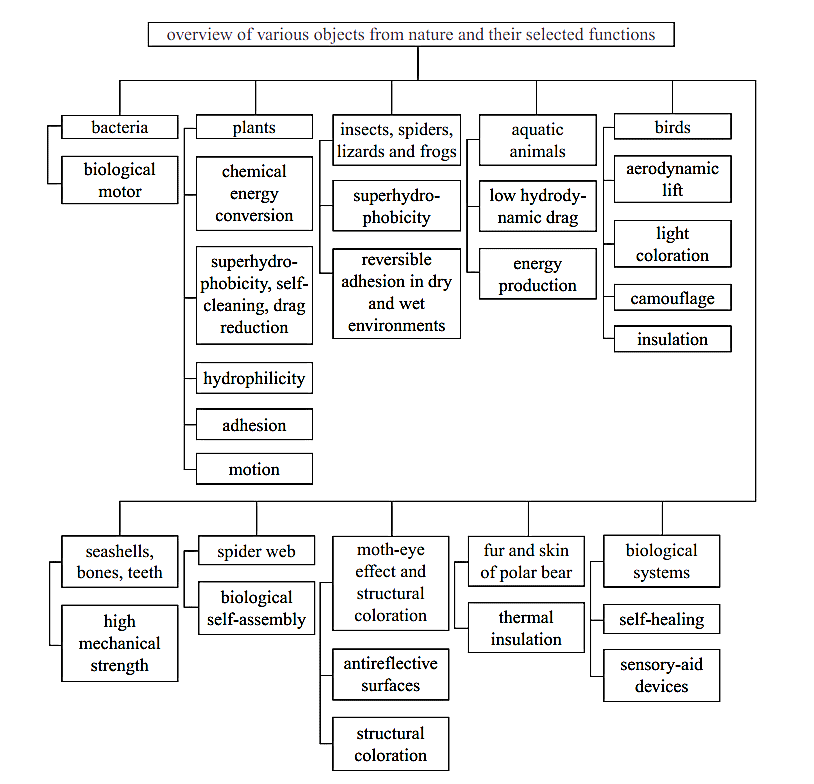
Few Examples of Biomimetics in Industry
- Aeroplane was result of flying technique of birds. Leonardo Da Vinci sketched birds flying technique as early as 16th century while Wright brothers, first successful aviators, modeled their plane’s wings according the birds.
- Design and function of fins, which divers use, was copied from the legs of water creatures like seals.
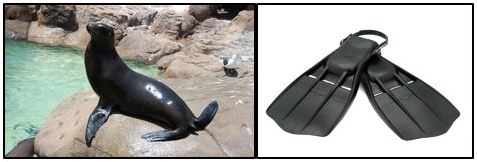
- Fastest train in the world, the Japanese Shinkansen Bullet Train, created too much noise when it emerges from a tunnel. Seeing how kingfisher dives to catch fish with little splash; the train engineers modeled the front-end of the train after its beak. The result is astonishing: a quieter train, with 15% less use of electricity and 10% faster speed [1].
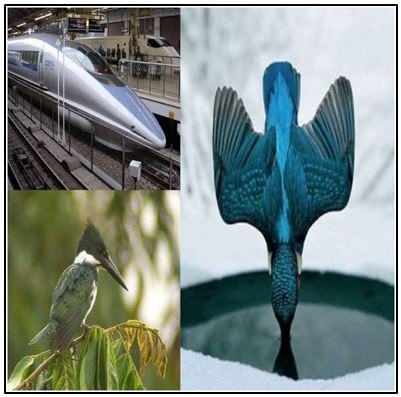
- Warning for Tsunamy war has been mimicked from extra sensory communication of dolphins which can sense at the very early stages.
- Gecko tape is a new invention by Prof A. K. Geim of University of Manchester, a glue-free, yet sticky, just like gecko. Geckos have amazing ability to move through all types of surfaces, vertically or horizontally at ease, which is due to the presence of billions of tiny fibers, ‘setae’ on their feet. These tiny fibers create Van Der Walls force with the surface, a weak force but billions of fibers make enough force to stick on the surface. No wonder we would see spider kids hang on the wall very soon.
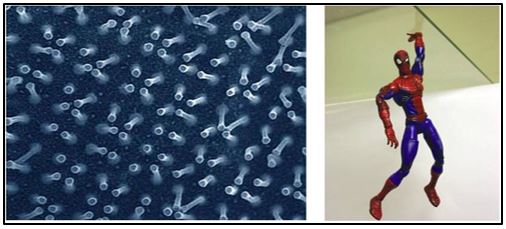
High Function Fibers Developed Using Biomimetic Approach
Discovery/Invention | Structures Mimicked | Advanced-Function Fibers | Discoverer/ Inventor |
---|---|---|---|
Lumen structure of cotton | Hollow fiber | Du Pont | |
Conjugate structure of wool | Elucidation of crimp structure Crimpled fiber | M. Horio (Kyoto University) | |
1964 | Silver trappings of leather | Artificial leather | O. Fukushima (Kuraray) |
1965 | Super-fine structure of leather | Micro-denier fiber Artificial suede | M. Okamoto (Toray) |
1978 | Micro-crater structure of cornea of moth | Fiber with deep colors and luster | S. Yamaguchi (Kuraray) |
1979 | Supramolecular structure of enzyme | Odor-killing fiber | H. Shirai (Shinshu Univ.) |
1980 | Triangular cross-section of silk | Shingosen with silk scrooping | Y. Sato (Toray) |
1980 | Capillary water absorption by tree | Water absorption porous hollow fiber | T. Suzuki (Teijin) |
1983 | Surface structure of lotus leaf | Water-repellent fabric | F. Shibata (Teijin) |
1989 | Multi-layered structure | Fiber with light interference function | K. Matsumoto (Kyoto Institute of Technology) |
Approaches of Development
Usually, there are Two Approaches of doing biomimetics
1. Top-down Approach
Engineers are searching for ways to optimize existing products or processes with the aid of biologists and their pool of biological knowledge. Because of the differing technical languages of those involved, it is very helpful if biologists acquire knowledge of various physical contexts, whereas engineers should be equally open-minded and willing to think in unusual directions.
After identifying the most promising solutions with additional, and sometimes very extensive, structural and functional analyses, biologists and engineers will abstract nature, deduce the functional principles, and create a modified and appropriate technical solution. Then engineers have to look for an optimal translation into techniques with appropriate production methods and materials. This strategy is also usable for optimizing already existing biomimetic technical solutions.
2. Bottom-up Approach
Biologists do fundamental research into nature’s structures, processes, and functional modes of operations. New principles are discovered and analyzed, then communicated to engineers, and the two groups work together to abstract and transfer insights into new technical solutions [4].
Functional Properties Introduced in Textiles by Biomimetics
Electrospun Superhydrophobic Structures Inspired by Nature
Nature has built a tremendous number of fascinating materials and structured surfaces with excellent surface wettability. Biomimetic investigation indicates that some natural phenomena, such as the self-cleaning effect of lotus and silver ragwort leaves ,the super hydrophobic forces exerted by a water strider’s leg , the anisotropic de-wetting behavior of rice leaves and waterfowl feathers, are all related to their unique micro- or nanostructures on surfaces.
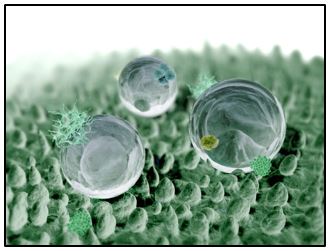
On the other hand, electrospinning has become one of the most popular methods for the fabrication of micro- and nanofibrous materials with controllable compositions and structures in recent years, and therefore offers excellent prospects for construction of biomimetic superhydrophobic surfaces. In this section, we will introduce the recent progress of some electrospun superhydrophobic structures inspired by nature.
Hierarchical Structures Inspired by Lotus Leaf
In nature, many plant leaves exhibit remarkable superhydrophobic properties. The self-cleaning lotus leaf is among the most well-known and studied examples, which exhibits a high WCA (Water Contact Angle) of around 161° & a small sliding angle about 2°.
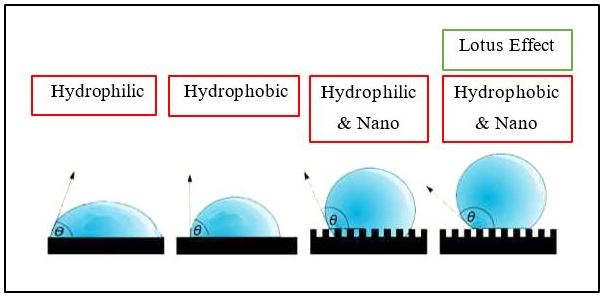
Raindrops are almost spherical on lotus leaf surface and able to roll off easily, which is usually referred to as the well-documented ‘‘lotus effect’’.
In 1997, Barthlott and Neinhuis firstly revealed the superhydrophobicity of lotus leaves.
Why Lotus Leaf shows this Superhydrophobicity?
Ans: Investigations showed that this unique property is caused by the surface micrometer sized papillae.
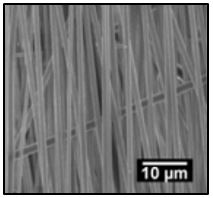
However, detailed scanning electron microscopy (SEM) images of lotus leaves (Fig. 2c) indicate that the surface of the lotus leaf is textured with 3—10 m size protrusions and valleys uniformly, which are decorated with 70—100 nm sized particles of a hydrophobic wax-like material.
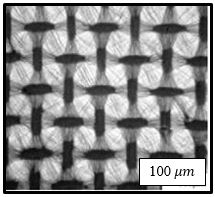
The cooperation of these special micro- and nanoscale hierarchical surface structures and hydrophobic wax-like material is believed to be the reason for the superhydrophobicity [5].
Inspired by lotus leaf, significant advances based on electrospinning technique now allow for the preparation of superhydrophobic surfaces.
Lotus Effect: Hydrophobicity and Self-clean in Action
- Leaves of the lotus plant are self-cleaning.
- Leaves are coated with nanoscopically tiny bumps and the bumps are covered with a thin layer of wax.
- Dirt particles have no grip on the surface.
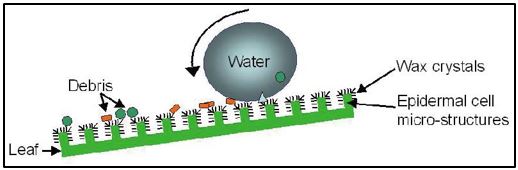
When it rains, water droplets roll down the leaves, pulling the dirt particles free and washing those clean [6].
Design of Anti-Dust, Water Repellent Fabrics (Inspired from Lotus Leaves)
Researchers have created superhydrophobic poly-lactic acid (PLA) fabrics via UV-photografting of hydrophobic silica particles functionalized with vinyl surface group over silica microstructure. Advantage of the technique utilized herewith is that such models can be successfully integrated not only in PLA but also in fabrics, exhibiting a robust method to design water and dust repellent fabrics.
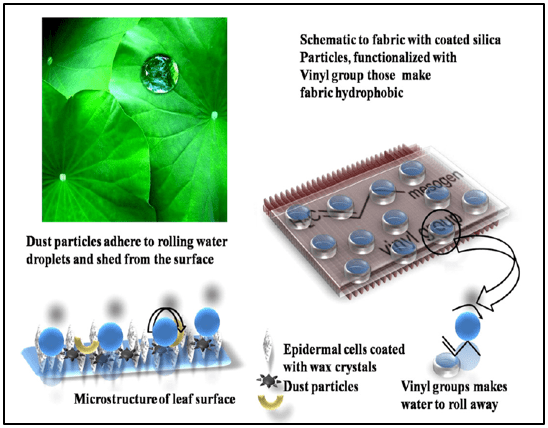
Spider Silk Inspired Anti-Tear Fabric Design
For a long time, the only natural source of continuous fibres, available in large quantities, was provided by the silkworms (Bombyx mori). The filaments were used for luxurious fabrics and technical applications (e.g., for parachutes) because of their qualities: smoothness, lightness, luster, softness, and strength.
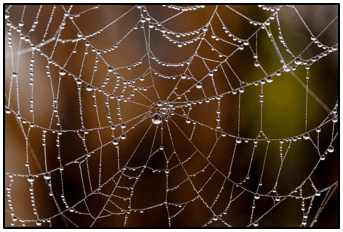
In addition, other insects too (such as butterflies, moths, and spiders) produce silk, but spider silk exhibits much better properties (unique combination of strength and elasticity).
The list with the remarkable properties of spider silk is very long, among which the most important characteristics are resistance (a yarn of one micrometer in diameter is five times stronger than a steel yarn of the same thickness), flexibility (stretches up to four times its length), and lightweight (a thread long enough to circle the globe on the equator would weigh 320 g).
Since spiders are difficult to keep in captivity (to harvest fibres), people have long studied them in order to mimic the principle by which they weave their webs. The spider web is composed of filaments of biopolymer (Polymeric substances occurring in living organisms) based on Protein (Keratin) which, although spun at ambient pressure and temperature and with water as solvent, presents outstanding mechanical properties due to semi-crystalline polymer structure [7].
Inspired by spider silk micro-nanocrystallite design, engineers are now able to model in the lab materials which have strength and stretchability similar to spider silk. The synthetic nano-reinforced structure emulating natural spider silk provides an opportunity to synthesize and conjugate polymer nanocomposites in future fabrics which will potentially rival the most advanced materials in nature.
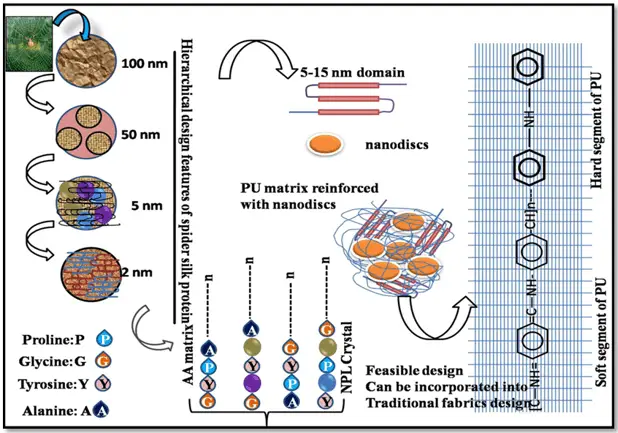
Using a new solvent-exchange approach that is amenable with current textile industry, engineers reinforce the hard micro domains of commercial polyurethane elastomer (a polymer or plastic consists of long chains composed of small repeating molecular units) with tiny clay discs (about 1 nm, or a billionth of a meter thick and 25 nm in diameter).
The interesting aspect of this reinforced molecular nanocomposite is that it can be easily tuned to make fibers similar to stretchy compounds such as Nylon or Lycra for traditional textile industry [6].
Gecko Inspired Dry Adhesion to Move Along Smooth Surfaces Vertically or Horizontally
Another recent discovery involves natural fibrous structures on gecko feet, which give them the ability to stick (dry adhesion) to and move along very smooth surfaces, often upside down.
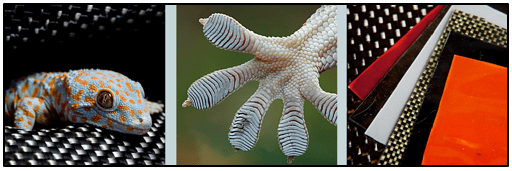
The skin on a gecko’s feet consists of a hierarchical structure of rows of setae, and spatulae. The footpad of a gecko is covered with very high density (about 5000/) of tiny fibres (setae). Furthermore, each seta branches into hundreds of spatulae with dimensions of approximately 100 nm (figure 16-b).
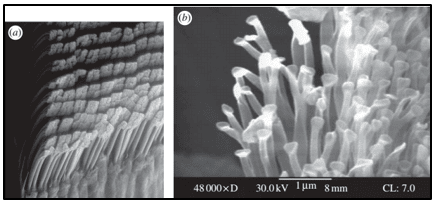
The complex structure uses a relatively simple mechanism of adhesion using van der Waals forces. Simply put, when two surfaces come in intimate contact with each other, considerable van der Waals forces can be generated. Results of direct setal force measurements attribute the adhesion to van der Waals forces rather than suction, friction or electrostatic forces.
The tiny fibre ends (spatulae) allow relatively unconstrained local deformation which is required to generate intimate contact with surfaces having local irregularities. Each gecko foot-pad seta can resist an average force of 20 mN, resulting in an adhesive force of 10N for a foot pad area of approximately 100.
Some gecko species have adhesion strength capabilities as high as 100 kPa!!!
So how Does Gecko Walk? Shouldn’t it be Impossible for it to even Move?
Ans: Although such strong adhesive forces would make the movement of the gecko difficult, this lizard has developed a unique way of walking by curling its toes for attachment and peeling during detachment to eliminate the forces between its foot and the surface, thereby enabling it to move with ease.
The task seems to be simple in that it requires fabrication of millions of tiny densely packed nanofibres standing up on their ends on a substrate much like flocked fabric surface. Needless to say, it turns out to be lot more complicated!!!
CHALLENGES
- Higher Aspect Ratio: The first challenge is to ensure that the tiny fibres are of sufficiently high aspect ratio in order to be able to make contact with the contacting surface, which is often microscopically irregular.
- Avoiding Entanglement & Bunching: The need for high aspect ratio leads to the second challenge in that the fine fibres tend to collapse and stick to each other leading to matting and entanglement. Additionally, if the spacing between adjacent fibres is too small, the intermolecular forces acting between the fibres lead to bunching. Theoretical analyses as well as experimental data point to the need for high modulus fibres of high aspect ratio with small inter-fibre spacing is required to achieve good adhesion.
Production Materials and Techniques
Synthetic gecko foot fibres have been created using various materials and techniques. These include, for example,
- Nanomoulding using silicone, polyimide, polyvinylsiloxane and polyurethane;
- Photolithography using polyimide, carbon nanotubes and polyurethane.
The reported adhesion strength in many of these cases exceeded that of gecko feet. Some of the most impressive results, arguably, are those obtained when Carbon Nanotubes are used as hairs. Independent reports by Ge et al. and Yurdumakan et al. claim that carbon nanotube-based gecko tapes can support significantly higher stresses than those supported by gecko feet [8].
Shark Skin Inspired Low Hydrodynamic Surface Drag
Most shark species (super order Selachimorpha) move in water with high efficiency and maintain buoyancy due to special ingenious anti-drag design of their skin which reduces drag by 5–10%. Scanning electron microscope studies have revealed the tooth-like scales of shark skin, called dermal denticles (little skin teeth or riblets) which are ribbed with longitudinal grooves (aligned parallel to the direction of local flow of water).
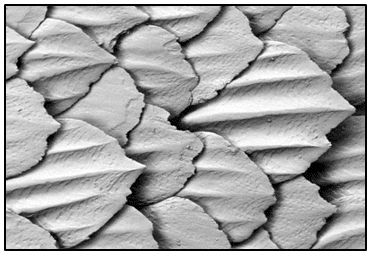
It produces vertical vortices or spirals of water, keeping the water closer to the shark’s body, thus, reducing the surface drag.
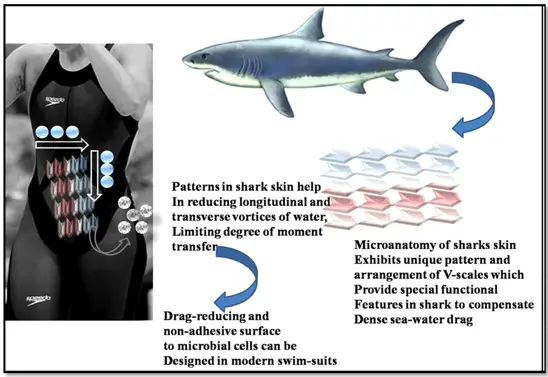
This fine microstructure varies from one location to another depending upon the body part participating in hydrodynamic balance while swimming. The V-shaped scales approximately 200–500 mm in height and regularly spaced (100–300 mm), are present over most of the shark’s body.
Moreover, this microscale longitudinal ridges influence the fluid flow in the transverse direction by limiting the degree of momentum transfer and the ratio of scale height to tip-to-tip spacing has critical role in reducing the longitudinal and transverse drags.
Another remarkable feature associated with this unique shark scale feature is its microtopography (The surface features of a material) that acts as antibacterial fouling surfaces and microorganisms find it inhospitable to attach on such grooved surface.
Inspired by natural design, scientists are adding technological improvements to swimming suits by designing antimicrobial fabrics without the chemical treatments. Especially in Olympic swimming competitions, 1/100th of a second can make the difference between winning and losing the event.
Now such important sport events are heading towards technological support since swimmers are using swimming suit designed on the hydrodynamics principles of a shark’s skin.
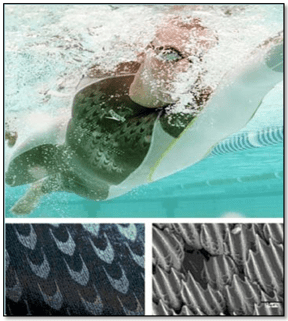
These tightly fitting suits, covering rather a large area of the body are made up of fabrics which are designed to mimic the properties of a shark’s skin by superimposing vertical resin stripes. This phenomenon is known as the Riblet Effect. Swimsuits made with the new fibers and weaving techniques mimicking shark scales microfeatures, are produced to cling tightly to the swimmer’s body. It may give the wearer a crucial equivalent head start in swimming competition by dampening turbulence in the immediate layer of water, next to the skin.
- SPEEDO swimsuit to mimic a shark’s skin. Known as FASTSKIN
- The idea is that water escapes along these channels.
Firefly Glow Designing E-Circuited Fabrics
Light production (glow) in fireflies arises on account of an enzyme catalyzed (luciferase) bio-chemical reaction called bioluminescence. This process occurs in specialized light-emitting organs, usually on a firefly’s lower abdomen (Fig. 7).
The enzyme luciferase acts on luciferin, in the presence of magnesium ions (), adenosene triphosphate (ATP) and oxygen to produce light.
This chemical process provides inciting motivation to design glowing clothes in dark that would add valuable assets to fabrics and textile industry.
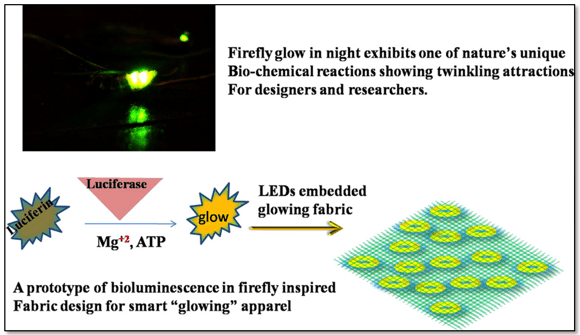
In this age of nano-innovation, researchers are able to produce at large scale the light-emitting devices with fabric printed circuit boards (PCBs) and successfully connect them with wearable display format using socket buttons. Thus, enabling firefly glow in fancy dresses, utilizing electronic textile engineering (e-fabric) design [6].
Hair with Hooks/ VELCRO
Many plant surfaces have hairs (also known as trichomes). The hairs are found
on the aerial surfaces of most flower plants, some conifers and mosses. The structures of these hairs are often complex. The function of the hairs
varies from one plant to another. Hairs might function as an anchor for seed dispersal by animals or wind. Some of the hairs form hooks for climbing purposes.
In Cynoglossum officinale, the hairs have lateral barbed hooks for seed dispersal.
Velcro Effect
Fruits of the burdock plant consist of hooks. Cockleburs get attached to clothes readily.
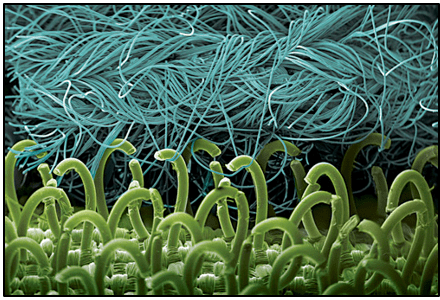
In 1948, Swiss engineer George de Mestal found that their spines were tipped with tiny hooks that stick out from the seeds and provide a hook-and-loop construction, which grips instantly, but can be ungripped with a light force. This led to the invention of a zip, called Velcro (1955), which works as a zipper. One side has stiff hooks as the burs and the other has loops in the fabric. The word Velcro was derived from the French words velour (velvet) and crochet (hook) [2].
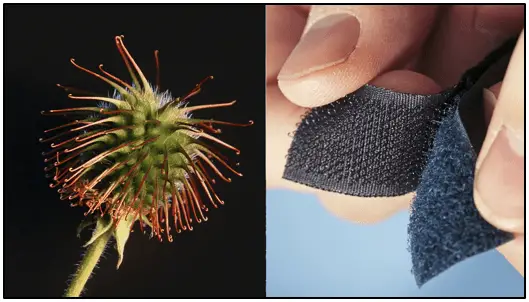
The commercial design was patented by George de Mestal in 1955 and set up a production site to manufacture sixty million yards of Velcro per year.
Today it is a multi-million dollar industry. Velcro has household, industrial, space program, and medical applications [3].
Touch Sensitive Apparel Design
Touch sensitive plant Mimosa pudica shows human muscle’s actin–myosin like quick sensing and actuation with its leaf-moving muscle, so-called pulvinus which performs touch sensitive hydraulic actuation.
Pulvini are swollen part at the base of Mimosa leaf stalks or petioles which act as autonomous organ, housing mechano- and photoreceptors that enables leave to move in response to external stimuli. Anatomically, all pulvini comprise thick walled, water-conducting vascular tissue, surrounded by thin walled motor cells.
These specialized cells undergo visible swelling and shrinking, actuated by changes in turgor pressure and rapid growth expansion across leaf epidermis involving ion transport as. This exhibits one of the remarkable weathering phenomena in plant tissue when touched and exemplifies the fastest plant movements.
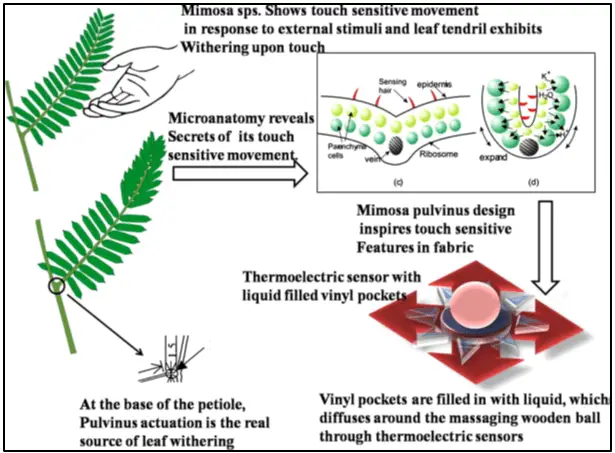
Mimosa pulvinus mediated touch sensitive actuation put forth an enormous opportunity to design fabrics which shrink and de-shrink in response to external stimuli such as touch, sound and/or light. In fashion industry, this would represent a dream opportunity to come true when models walking on ramp will show folding–unfolding modes of smart fabrics with novel sensing capacity.
Adopting functional mimesis to the Mimosa leave pulvinus, researchers have designed haptic fabrics by knitted smart materials with touch therapy features. Such wearable fabrics equipped with actuators and sensors perform artificial massaging and aromatizing functions while walking.
Most important, such fabrics could provide a sympathetic side of apparel design by attending, understanding and responding to another person’s emotional expressions, a fundamental requisite of elderly person, spending lone time in hospitals.
Pine Cone Inspired Hygroscopic Movements to Design Smart Breathing Fabrics
The scales of seed-bearing pine (Pinus radiate) cones move in response to changes in relative humidity. This hygroscopic movement is motivated by a structural–functional mechanism at the base of each seed petal or scale (that houses the developing seed) of the pine cone.
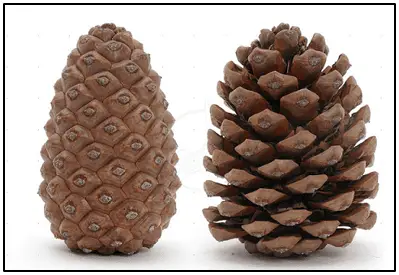
When dries, it automatically opens up by moving away the scales gape, facilitating release of the cone’s seed. Whereas when it is kept in moist (damp) environment, scales close up. Microscopic anatomy of Monterey sps. cones reveal two types of scale growing from the main body of the cone –
- The Ovuliferous Scale
- The Bract Scale
The larger ovuliferous scales bear microscopic sclerenchymatous (cellulose) fibers on upper and lower surface (8–12 mm in diameter, 150–200 mm long). It respond to changes in relative humidity by opening–closing cone aperture when season changes.
In addition, orientation of cellulose microfibrils between two layers of scales and their expansion in response to relative humidity further controls the vital bending of the scales, facilitating opening–closing of the cone aperture for seed dispersal.
Development of Smart Breathing Fabrics
This natural phenomenon inspired the researchers to mimic the pine cone for designing humid sensitive adaptive cloth, delivering relief from the discomfort caused by moisture in clothing microclimate as experienced in urban environments.
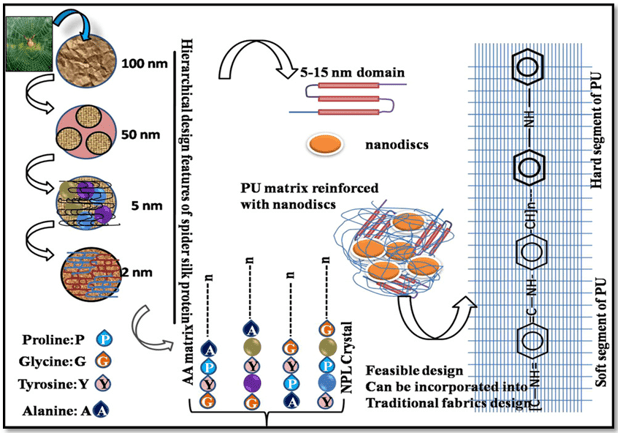
The fabric design utilizes Two Layers: one of thin spikes of wool, water absorbent material which opens up when gets wet by the wearer’s sweat, like ovuliferous scale in pine. When the layer dries out, the spikes automatically close up again.
An underneath second layer protects the wearer from the rain and this smart fabric works like breathing cloth, taking dry air in while closing the fabric pores and moist air out while opening.
Such fabric could adapt to changing temperatures by opening up when warm and shutting tight when cold, just like a pinecone’s bract.
Camouflage
Camouflage is the use of any combination of materials, coloration, or illumination for concealment, either by making animals or objects hard to see (crypsis), or by disguising them as something else (mimesis).
The phenomenon of camouflage in certain fishes and amphibian occurs due to excellent iridescent lateral stripes or spots which change their color from blue–violet under low light to green, orange and/or red under increased light intensities.
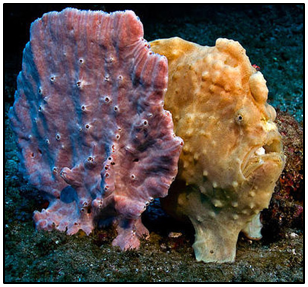
The reflected iridescent colors are produced by the constructive interference of light from the stacks of thin alternating transparent layers with different refractive indexes.
How Chameleon Changes its Color?
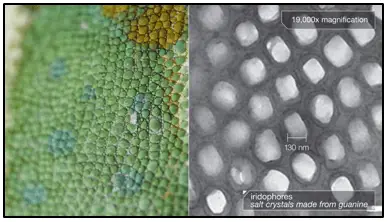
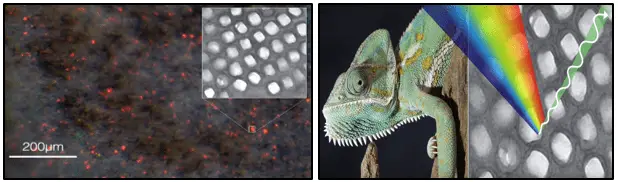
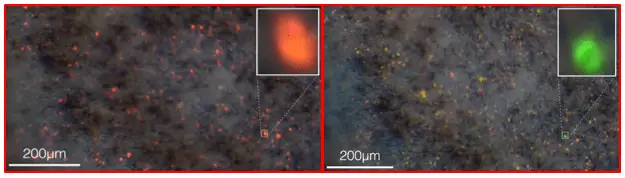
Development of Camouflage Skin & Apparel
1. Electronic Skin
In the following, we can see bio-inspired stretchable e-skin with interactive colour-changing and tactile-sensing properties. This concept is realized through the development and integration of a stretchable highly tunable resistive pressure sensor (PS) and stretchable organic electrochromic devices (ECDs).
This e-skin, besides detecting applied pressure, is also able to distinguish varying applied pressures through real-time visible colour change. This work demonstrates low power consumption, interactive and colour-changeable e-skin, which is readily prepared by a cost-efficient all-solution processing approach.
![A Chameleon-Inspired Stretchable Electronic Skin with Interactive Colour Changing Controlled by Tactile Sensing [10]](https://textiletuts.com/wp-content/uploads/2020/01/A-Chameleon-Inspired-Stretchable-Electronic-Skin-with-Interactive-Colour-Changing-Controlled-by-Tactile-Sensing-10.png)
2. Camouflage Apparel
Inspired by this nature’s cryptic phenomenon, scientists have designed choleric liquid crystals (CLCs) to alter the visible color of an object to create the thermal and visual camouflage in fabrics. The color of CLCs can be changed with temperature sensitive thermocouples.
The heating–cooling ability of thermocouples can be used to adjust the color of the liquid crystals to match the object’s background color, providing camouflage or adaptive concealment as schematically depicted below.
Moreover, nature- inspired camouflage in animals has stimulated optical camouflage research in fabric design to develop and impregnate the phased optical array (OPA) like holographic designs in three dimensional hologram of background scenery, on an object to be concealed.
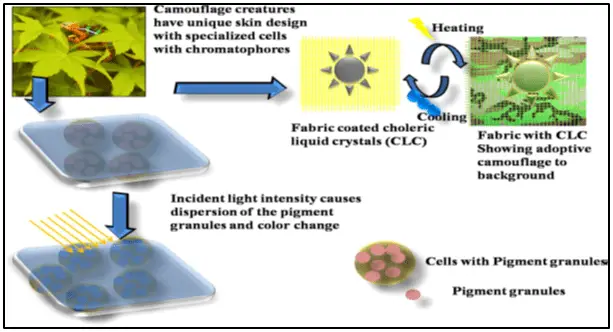
Self-Healing Fabric
Self-healing fabric design inspired by nature’s healing mechanism in mammalian tissue. Nature’s self-healing ability has inspired new ideas and mechanism which are of fundamental interests for the engineers in designing self-healing fabric.
Healing process in mammals is much complex and involves hemostasis (arrest of bleeding), inflammation (recruiting immune cells to clear of any microbial population and cell debris), proliferation (growth of new tissue), and remodeling (retaining tissue shape like before injury).
All these events take place spontaneously and autonomously in ordered phases, triggered by injury processes at wound site but healing process is time consuming. Moreover, in mammals, the intrinsic mechanism of healing evolved around the chemical reactions of a series of active enzyme cascades and their inactive precursors, known as clotting factors.
An important aspect of the mammalian healing is the rapid hemostatic response to arrest the bleeding and thereafter actual tissue and skin healing starts which is a more lengthy process. In mimicking bio-inspired self-healing program, a reasonably rapid response is required to restore the degree of structural integrity or prevent crack propagation while applying it to fabric design.
In addition, mimicking such enormously complex and lengthy process for smart fabric design has limitations due to the lack of replenishment of the engineering components in the system designed for self-healing fabrics.
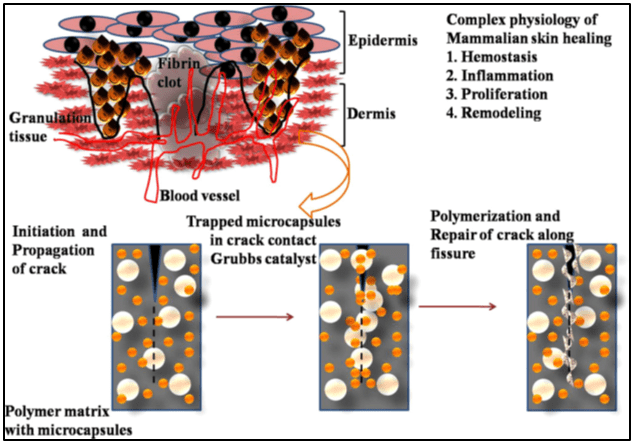
Self-healing Capable Polymer Composite
Nonetheless, nature’s healing machinery has inspired chemists/ engineers to propose new ideas and mechanisms with different healing concepts which offer the ability to restore mechanical performance of materials via fusion of the failed surfaces. Using biological bleeding approach to healing, White et al. has created the microcapsules reinforced with hollow fibers, polymer composites. This lightweight material exhibits high stiffness and superior elastic strength over the conventional materials.
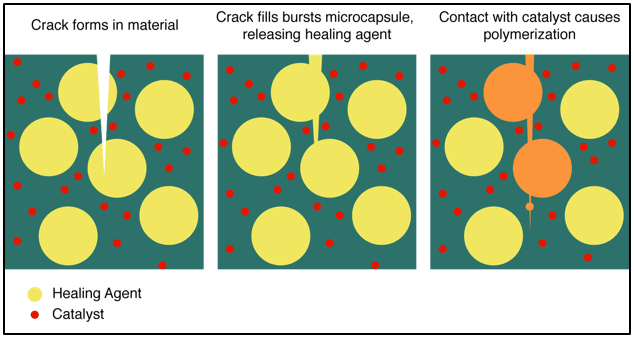
Microencapsulation of self-healing components involves the use of a monomer, dicyclopentadiene (DCPD), stored in urea–formaldehyde microcapsules dispersed within a polymer matrix.
When microcapsules are ruptured by a progressing crack, monomer is drawn along the fissure where it comes in contact with a dispersed particulate catalyst (ruthenium based ‘Grubbs’ catalyst), initiating polymerization, thus repair the crack. Release of active components has clearly shown the restoration of the lost mechanical properties arising from the macroscale crack within a polymer matrix.
Moreover, results confirmed that the dispersion of microcapsules within composite laminate material was not detrimental to stiffness of the parent architecture. A notable advantage of the microencapsulation self-healing approach is the ease with which it can be incorporated into a bulk polymer material which could be a potential self-healing reinforcement agent for the future fabrics.
In another landmark approach to design smart fabrics, the self-healing rubber like material has been designed which acts as molecular glue and seals the lost end when two broken pieces are brought together.
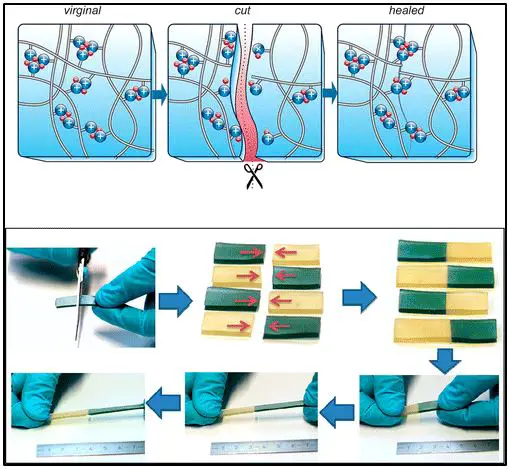
This thermosensitive polymer material has been made by simple supramolecular chemistry of fatty acids and urea, provides an exciting opportunity to be incorporated into fabrics [6]. This rubber can spell the end of punctured tires!!!
Conclusion
Nature is an extremely vast database of structures and mechanisms that proved to be clearly superior to those man-made. There are numerous examples of fibrous structures, multifunctional materials, thermal insulating materials, structural colours, and many others that can serve as sources of inspiration for future sustainable textiles.
In many ways, textiles offer unique opportunities to imitate nature. The base units of each textile structure at the most elementary level of the hierarchy (from nano to micro) are organic fibres, many of which are natural.
In addition, like many natural functional surfaces, the textile surfaces also offer excellent opportunities for developing new functionality. All these allow an easier way to borrow the biomimetic principles of nature in textile field than in other industrial areas.
REFERENCES
Bar-Cohen Y. Biomimetics? using nature to inspire human innovation. Bioinspiration & biomimetics. 2006;1(1):P1.
- Bhushan B. Biomimetics: lessons from nature–an overview. Philosophical Transactions of the Royal Society of London A: Mathematical, Physical and Engineering Sciences. 2009;367(1893):1445-86.
- Uddin DMA. Biomimetics: Engineering New Textile [18/08/16].
- Milwich M, Speck T, Speck O, Stegmaier T, Planck H. Biomimetics and technical textiles: solving engineering problems with the help of nature’s wisdom. American Journal of Botany. 2006;93(10):1455-65.
- Wang X, Ding B, Yu J, Wang M. Engineering biomimetic superhydrophobic surfaces of electrospun nanomaterials. Nano today. 2011;6(5):510-30.
- Lavate S. Biomimetics in Textiles [18/08/16].
- Teodorescu M. Applied Biomimetics: A New Fresh Look of Textiles. Journal of Textiles. 2014;2014.
- Eadie L, Ghosh TK. Biomimicry in textiles: past, present and potential. An overview. Journal of the royal society interface. 2011:rsif20100487.
- Nature’s Mood Rings: How Chameleons Really Change Color [18/08/16].
- Chou H-H, Nguyen A, Chortos A, To JWF, Lu C, Mei J, et al. A chameleon-inspired stretchable electronic skin with interactive colour changing controlled by tactile sensing. Nature Communications. 2015;6:8011.